VentureBeat: This did make we wonder if we could just store information in our bodies, though.
Sandhu: If you want to go back 15,000 years, in the Indian Vedas — I’ve been reading up on this lately, so I’ll bore you for just a moment — those speak of our body having eight different types of our memory. The memory we store in our brains, in our mind, what we pick up as we learn over time, is only one of those eight. Their point was, our body–for example, the skin, the features on your face, that’s actually a memory of your forefathers, for however many generations you want to go back. Our body stores a lot of information, but over a period of time. We call it evolution, the features we pick up as the generations go by. So in a way what you’re saying is true, and even more than you might realize.
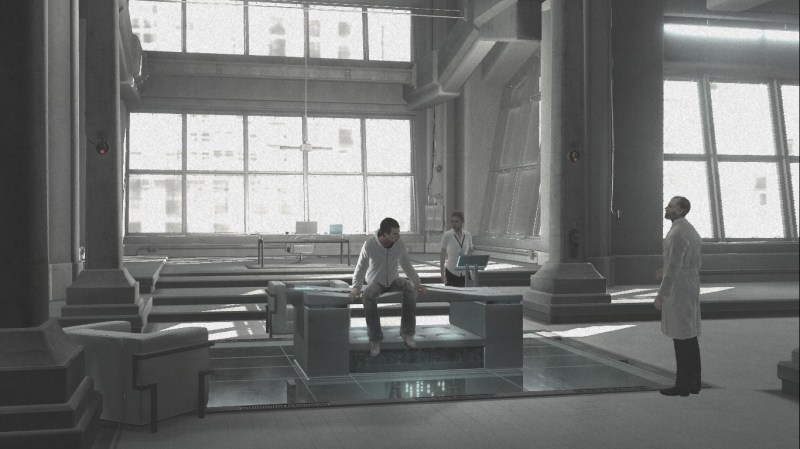
Above: Abstergo Industries’ Animus machine lets you travel back in time to your ancestor’s DNA memories in Assassin’s Creed.
VentureBeat: There’s a famous video game called Assassin’s Creed. The premise is that you can go back in time through your ancestor’s DNA and relive their memories, their lives.
Sandhu: Yeah, I’ve seen my kids play it. Even modern science backs this sort of thing up now. Only four percent of your DNA actually seems to be programming your body. The rest of the DNA just sits under that four percent. But we don’t know how to manipulate this information. What we can manipulate and learn is the information in our brains. That’s not necessarily DNA storage, but long-term memory, over evolution, over generations, that could be a similar concept.
June 5th: The AI Audit in NYC
Join us next week in NYC to engage with top executive leaders, delving into strategies for auditing AI models to ensure fairness, optimal performance, and ethical compliance across diverse organizations. Secure your attendance for this exclusive invite-only event.
What looks like science fiction is sometimes borne out once we learn more about the science. Sometimes it’s only fiction, and sometimes–the Vedas also talk about 31 dimensions of the universe, and string theory is up to 13. They’ve solved equations up to 13. Is it going to reach 31 eventually?
VentureBeat: How have people reacted to this, whether your colleagues at Micron or other scientists?
Sandhu: DNA always appeals to our imagination. It’s such an important part of us. The human body is the most sophisticated and complicated machine ever built, whoever built it. None of our computers, in complexity and capability, come close. Our brain processes a humongous number of transactions every second, based on our best estimates. Since DNA is part of us, we should appreciate it, I suppose.
In the technology side of things, we’ve tried to work with polymers and so forth, which technically speaking are similar molecules. The fundamental bonds are hydrocarbons in both cases. Hydrocarbon bonds are very difficult to work with, because they’re not very stable. But here is this DNA material, which has very similar fundamental bonds–this is a big part of why we did the study, because I was very intrigued. How can the same hydrocarbon bonds, in the form of a DNA molecule, turn out to be so stable?
What comes out is, your DNA–as you know, it folds and unfolds as part of the process of normal life. It has to work within a certain temperature range. We’re pretty fragile in that sense, with a narrow temperature range. But DNA folds and unfolds and can change the stability of the molecule based on its configuration. In your body, DNA has to be easily manipulated when cell division is going on. It has to unfold and split into two very easily. But then, once that cell turns into your skin or whatever, it has to be very stable again.
So DNA is able to perform functions that are pretty amazing. It can be both unstable and stable based on its configuration, even though the fundamental hydrocarbon bonds are the same. Our study showed that all this DNA can be stable for hundreds of years, even though it can also perform millions of cell divisions in our bodies each day.
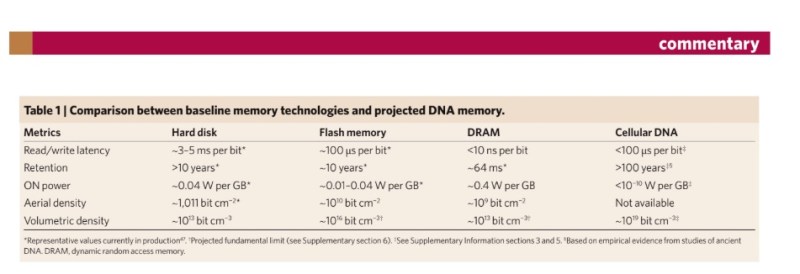
Above: DNA could have an edge on other memory types.
VentureBeat: We’re all familiar with evolution and mutation. Does that play in to what you’re working toward? Can it help you or hinder you?
Sandhu: Before I answer that question, one other thing I found very intriguing–your body has so many cell divisions going on every time. There’s a natural self-repair mechanism going on in that process. With millions of divisions going on, and environmental factors — light, cosmic rays, all these things — a very high percentage of these events have an error. The split doesn’t go as planned. There are some estimates that, based on our error rate, our bodies should simply disintegrate in less than 24 hours.
Nature has something called self-repair in our bodies, working in the background. Every time something goes wrong, your body corrects for it or compensates for it. That piece is pretty important. So, going back to your question on mutation, that happens over generations. As people have shown through the study of evolution, it happens in reaction to your environment. That’s a long-term process that happens for survival. If you move from a tropical place to someplace cooler or vice versa, over time people with darker skin are selected, or something like that. Mutations are favored and people start looking in a certain way.
The short-term errors I talked about and then longer-term mutations, you could say they’re linked in some ways. Whatever survives the most in a given environment over time, that’s evolution, or a selection process. But it’s the same fundamental mechanism in the DNA that drives both. In the case of memory systems using DNA, there’s also a notion of self-repair and error correction methods that we can apply to keep our information intact.
VentureBeat: Our bodies have error correction, then.
Sandhu: They do, or self-repair. Self-repair is an active process, which I found very amazing. Error correction is just something where you say, “Okay, this is wrong, I reject this piece of information.” Which is important, but to be able to find an error and repair that–we have ways to repair as well, in a consistent way. That’s pretty interesting. Our body ends up repairing itself.
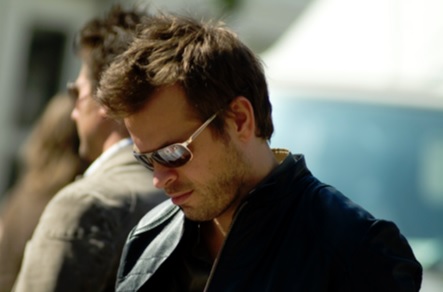
Above: Can we be Brad Pitt?
VentureBeat: Why don’t I get fixed to the point where I look like Brad Pitt, then?
Sandhu: [laughs] Nature still does its own thing. Everybody being Brad Pitt might not be the best outcome for humanity.
VentureBeat: How do you proceed from here? How much support do you need for this as far as grants or other ways of moving forward?
Sandhu: There’s always excitement any time you discover something new. We have a new group of people working on this. But from our perspective we have to look at the larger piece around how this will become practical, and what might be the challenges in getting there. Once we identify the challenges, we’ll try to encourage research and work in that space so we can solve some of these problems.
My goal is to make sure the physics is right. Once I’m convinced of that, there’s a potential in this medium. Now the question is, how do we make it real? There are all sorts of claims, but from our business, we know how memory systems work, and we look back at the history a little bit. We participate in the SRC consortium, which is funding some of this work. IARPA is involved in funding one of the projects in conjunction with SRC. IARPA, as you know, are the same guys who invented the internet, funding research into that.
A couple of years after we published our paper, we’re seeing other companies — Microsoft and others — a lot of people paying attention to this. Once you have that type of activity going on, hopefully the focus shifts into making this real. Cost, performance, making the system work. The starting point of this is archival memory. That’s where you have hard disks, or tape drives. It turns out that tape drives have to be rewritten every five or 10 years. It’s not very persistent memory. With the type of information we’re generating these days, rewriting those things is a big part of their cost now, if you look at it over 100 years and beyond.
We’re producing so much information now compared to before, and we want to store it forever. In order to store it forever, you have to rewrite it, and that cost is significant in terms of energy. That’s one of the interesting possibilities for DNA. If something can stay stable for 100 years, as opposed to five years, so you don’t have expend energy maintaining it–the other part, if you run the numbers, a teaspoon of DNA could contain all the information we know today about our universe. It can hold that much information. The form factors are pretty attractive. The challenge is just how you read and write into it.
That’s where the focus is now. You have to design systems and come up with a way to read, write, and manage this information, and then target specific applications of interest. That’s why we’re helping direct this research funding. The IARPA project I mentioned, which we’re part of, and SRC as well, is right on the money. It’s focused right on those things. It’s been good to see the synergies we have. There are opportunities to expand further from there, too. In a way this is just the start.
The problem, as I say, is pretty basic. We’re creating so much information, and storing that over long periods of time is a challenge. We see that getting exponentially worse over time.
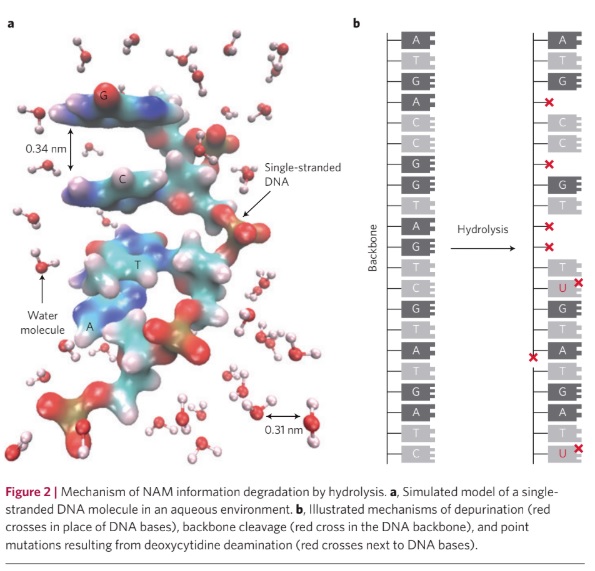
Above: How DNA memory may need error correction.
VentureBeat: How much activity like this is going on at Micron? Is it a large department, or fairly small?
Sandhu: Looking at different types of memory for the future is a pretty big activity. I was doing DRAM in the ‘90s, and in the early 2000s I went to NAND, when the iPod happened and the whole NAND thing exploded. I was on the early side of those things. We’ve had a find ways to get ahead. Then, in 2006 to 2008, I started this whole–what comes after NAND? What else is out there? We’ve looked at many different applications, many other types of memory — spin torque RAM, phase change, all kinds. We looked at every mechanism out there we know. Each time we looked at the physics of it, and then what kind of system we could build, and what would be the end market.
Out of that activity, DNA is the latest one we’ve identified. From those previous activities, there are some new memories we’ve put in the market. You’ve probably heard of 3D Xpoint under the different names we call it. DNA is now the latest thing, and it’s potentially an archival memory space. But it really challenges your imagination. It could go so many different ways if we could master the engineering side of things.
It’s a paradigm shift any way you look at it, but there have been similar shifts in the past. They look normal now because we read about them in books, but the background behind it, if you consider what it felt like at the time, it was pretty revolutionary. The whole silicon chip revolution, we went so many directions before we got here.
This is interdisciplinary in many ways, even more so than it was in the past. The disk drive was magnetics and then micromechanical systems. Those were different fields of engineering and science. This one challenges us even further. It goes into chemistry and biochemistry, and maybe even health sciences in some ways. On the other side, you still have to get the information into a computer. The chip side of things, the electronics side of things, they all have to be considered in the system. How do you merge this in a way that it all talks to each other and you can build a system out of it?